Compressible Turbulence: From Cold Atoms to Neutron Star Mergers
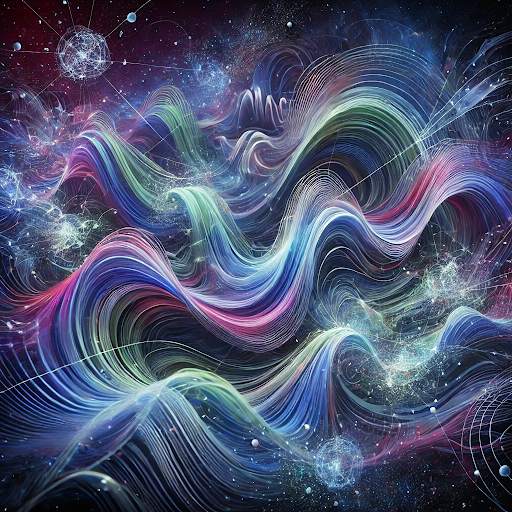
Event ID: INT-25-2a
Embedded Workshop dates: June 23 - 27 (Note: For workshop participants, there is a required $75 registration fee).
OVERVIEW
Turbulence in compressible fluids is at the forefront of current hydrodynamic research with applications that include supersonic flight, combustion, fusion, ultra-cold atoms, and nuclear astrophysics. While much more common in the Universe than incompressible turbulence, it is significantly less well understood due to its large parameter space, complex hydrodynamic-thermodynamic couplings, and difficulty in experimentally realizing relevant parameter regimes. Despite fixing the density to greatly simplify the mathematical formulation, incompressible turbulence still pushes the limits of current computational abilities. Compressible turbulence is even more difficult and needs a combination of inputs from both theory and experiment.
Quantum simulators in the form of ultra-cold atom experiments, provide a new platform for studying compressible turbulence. Bose and Fermi gasses can be manipulated with remarkable precision to simulate a wide variety of physical scenarios, providing table-top experimental access to regimes difficult to access with other techniques. This program will bring together both theorists and experimentalists to explore many aspects of compressible turbulence, with an embedded experimental workshop to elucidate what insight quantum platforms can provide.
PHYSICS APPLICATIONS
Some of the physical applications explored in this program are:
Nuclear Astrophysics: Quantum turbulence exhibits similarities with classical turbulence leading to the hypothesis that classical hydrodynamics will emerge from quantum systems after suitable coarse graining. Understanding the form and accuracy of such macroscopic hydrodynamic frameworks is crucial for understanding the impact of microscopic quantum turbulence on macroscales, such as glitching phenomena in neutron stars and likely also to the dynamics of neutron star mergers as well.
Pulsar Glitches: Glitches in the rotation rate of neutron stars are supposed to happen when a differential rotation builds up between the outer crust and an interior superfluid. Vortex pinning is conjectured to be responsible for this, and spin-up experiments in superfluids may help validate this picture. On the other hand, classical turbulence also has a propensity for sudden accelerations followed by gradual decelerations (and vice versa) which momentarily produce an imbalance that appears to have nothing to do with superfluidity. Are these manifestations of the same phenomena, or independent and incompatible effects?
Relativistic Turbulence: Compressible turbulence also plays an important role in other astrophysical contexts, including neutron star mergers and supernovae explosions. These applications require relativistic hydrodynamics which may also be simulated in cold atom experiments by making use of the approximately linear phonon dispersion resulting from the Nambu-Goldstone theorem. Here phonons play an analogous role to photons, allowing one to study phenomena like Hawking radiation from sonic black holes, coherent phenomena in galaxy formation (axion BECs), or using expanding clouds to study cosmology and curved space-time. More directly, compressible magnetic turbulence has been conjectured to explain high energy cosmic rays.
Hypersonic Hydrodynamics: The low speed of sound in cold-atom systems enables one to model supersonic phenomena like shockwaves and related turbulence. This addresses another interesting problem related to the microscopic nature of turbulence. The standard thinking in classical turbulence is that even the smallest scales of motions are far enough away from the microscopic time and length scales of molecular motion that the possibility of direct connection can be ignored. This cannot also be true in hypersonic flows where the translational motion is very fast so that the internal structure of the fluid motion becomes quite important for turbulent dynamics and is certainly not true in quantum turbulence. Thus, a case exists for studying microscopic theories with the intention of discovering proper macroscopic theories more general than Navier-Stokes equations.
If you have thoughts, ideas, or results about compressible quantum turbulence, related applications, or how compressible quantum turbulence relates to other turbulent phenomena, please apply to this program.
Numerical simulation of compressible turbulence in a unitary Fermi gas on a 1003 cubic lattice. A perturbed interleaved vortex–anti-vortex lattice decays through quantum turbulence through the crossing and reconnection of vortices. Compressible systems also permit wave turbulence through sound waves, the interplay of these mechanisms will be one of the topics discussed in this program. This type of superfluid can be studied in cold atom experiments, but is a good model for the dilute neutron superfluid in the crust of neutron stars.
For details see: Wlazlowski et. al, "Characterizing the cascade of energy in fermionic quantum turbulence: Pushing the limits of high-performance computing", PNAS Nexus 3, p. 160 (2024), https://doi.org/10.1093/pnasnexus/pgae160